Ethylene-based plant growth regulators (PGRs) for mitigating spring frost injuries in stone fruit species
September 10, 2020
Ethylene-based plant growth regulators (PGRs) for mitigating spring frost injuries in stone fruit species
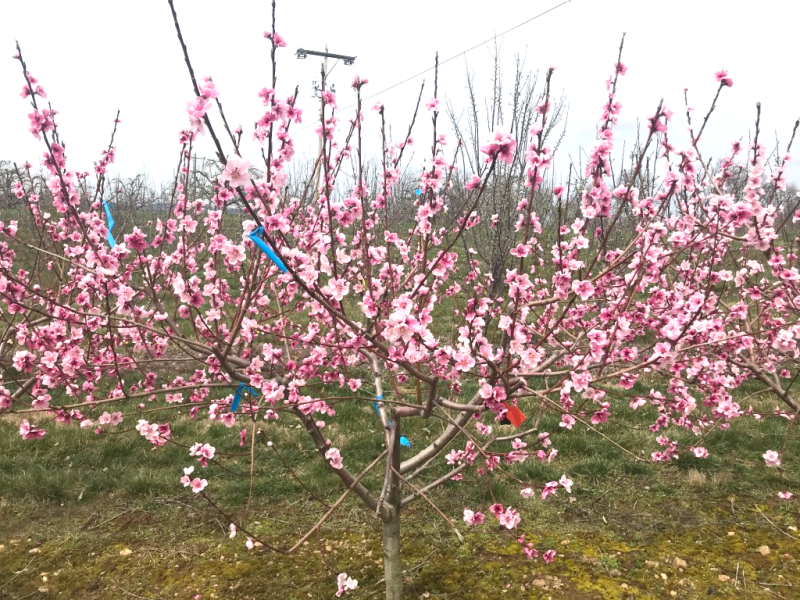
The sustainable annual production of stone fruits in Virginia and other fruit-growing states is constantly imperiled by spring freezes. Economic losses caused by frost damage can be enormous, and the risk of frost damage is projected to rise as a result of global climate change. Global warming increases the risk of frost damage by advancing the timing of bud break and flowering and/or increasing the frequency of “false spring”, thus making frost damage a worldwide challenge in fruit production. This was reflected in a survey conducted in 2017, in which 67% of fruit growers and stakeholders in Virginia ranked the need for research on strategies to avoid or reduce frost damage in apple and peach as being important or very important. The available frost mitigation approaches (e.g., under-tree sprinklers, wind machines, helicopters, and gasoline heaters) are either ineffective, labor-intensive, economically inefficient or environmentally unsustainable. One of the avoidance mechanisms that has been suggested, yet to be accomplished, is to delay bud break and bloom date in the early-bloom species and cultivars beyond the last day of frost. Toward this goal, several studies have investigated the applicability of plant growth regulators (PGRs) as foliar applications to delay bud break. Ethylene is one of plant hormones that affects the progression, maintenance and release of bud dormancy in deciduous woody perennials, including pome and stone fruits (Figure 1). Moreover, ethylene has been used on fruit trees for decades to delay bloom and avoid frost damage. Ethephon is a plant growth regulator that degrades and releases ethylene once entering plant cytoplasm. Many studies have reported that fall application of ethephon can effectively delay the blooming time in the following spring in many fruit species, especially stone fruits (Table 1). Despite the significant success in using ethephon to delay bloom and prevent spring frost, some studies have indicated beneficial effects of ethephon can be comprised by the occurrence of detrimental effects such as gummosis, leaf yellowing and abscission, terminal dieback, flower abscission, floral bud failure, low fruit set, and yield reduction (Table 1).
Dr. Jianyang (Jay) Liu, a research associate in Dr. Sherif’s lab investigates concentrations and application timings of ethephon that can delay bloom in peach and sweet cherries cultivars. The effects of ethephon on chilling and heat requirements, leaf abscission rate, cold hardiness, bloom delay, crop yield and tree injury have been assessed in two peach (Red Haven and Sun High) and three cherry (Regina, Skeena and Selah) cultivars grown at the AHS Jr AREC, Winchester. Preliminary results confirmed the effects of ethephon in delaying bloom date in peach, and such effects were dependent on application timing and concentration (Figure 2). Transcriptomic analyses and hormonal profiling will be also performed on buds treated with ethephon to quantitatively characterize hormones, hormonal pathways and molecular networks that are altered by exogenous ethephon applications during bud dormancy.
Figure 1: Proposed schematic model of hormone interactions during bud dormancy induction and release in woody perennials. Solid arrows and lines indicate actions or interactions among hormones, pathways, and environmental cues that have been documented in the literature. Red color indicates the substance or process that induces dormancy, and green color indicates those that promote dormancy release. Both SD photoperiod and low temperature induce CBF, which directly promotes the expression of DAM genes. DAM proteins regulate dormancy through ABA-dependent and ABA-independent pathways. In the former pathway, accumulation of DAMs reduces GA level, thus activating DELLA and subsequently XERICO proteins, which in turn promotes ABA synthesis. DAM genes can also upregulate ABA levels through upregulating the gene encoding NCED, a key enzyme in ABA synthesis. In addition to the control of DAMs over ABA biosynthesis and signaling, ABA can also negatively regulate the expression of DAM genes through SnRK2 and the ABA signaling component AREB. This pathway probably serves as a negative feedback regulation mechanism. In the ABA-independent regulation pathway, DAM proteins induce dormancy through negative regulation of FT, which in turn prevents CCG-mediated dormancy release. ABA can also repress CCG and inhibit the mitotic cell division. ABA was also found to suppress the intercellular communication during dormancy by enhancing the expression of callose synthase, leading to callose deposition and blockage of plasmodesmata. This alteration of plasmodesmata during dormancy is reversed by GA as buds transition to bud break. GAs induce the expression of glucanases which degrade callose, allowing for the passage of sugars and other growth-promoting factors. Under SD conditions, GA biosynthesis is inhibited through phytochrome and phytochrome-interacting factors. ET is induced by SD and has a negative impact on GA biosynthesis and signaling, which nominates it as a dormancy inducer. However, ET also inhibits ABA synthesis and signaling and negatively regulates CBF through activation of EIN3, which is also subject to the regulation of JA through JAZ proteins. JA induces bud dormancy by targeting JAZ proteins for degradation via the ubiquitination/26S proteasome, which in turn releases MYC2 and ICE1 from repression. Both MYC2 and ICE1 activate the expression of CBF. CK can repress ABA and promote dormancy release through inducing the expression of CCGs. IAA facilitates dormancy release through promoting GA biosynthesis and callose degradation. ABA, abscisic acid; CBF, C-repeat binding factor; CCG, cell cycle gene; CK, cytokinin; ET, ethylene; EIN3, ETHYLENE INSENSITIVE 3; FT, flowering locus T; GA, gibberellins; JA, jasmonates; IAA, indole-3-acetic acid; SD, short day; NCED, 9-cis-epoxycarotenoid dioxygenase; DAM, dormancy-associated MADS (Liu and Sherif, Frontiers in Plant Science, https://pubmed.ncbi.nlm.nih.gov/31620159/)
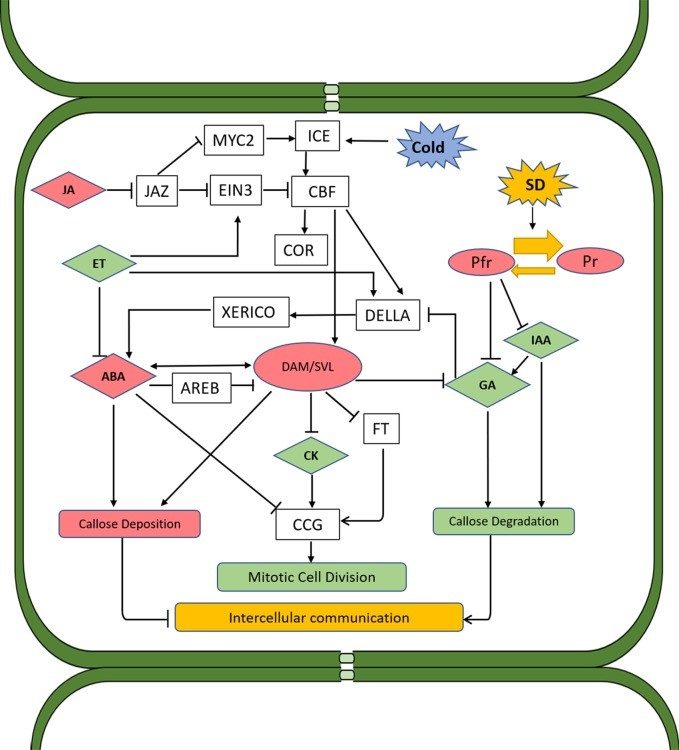
Link to Liu and Sherif, Frontiers in Plant Science https://pubmed.ncbi.nlm.nih.gov/31620159/
Table 1. Effects of Autumn-Applied Ethephon on Fruit Trees Note: Yield reduction which was masked and confounded by the occurrence of spring frosts is labeled as year effect, and data not available, NA. Asterisks (*, **) refer to defoliation stage (time and %, respectively) when ethephon was applied. For the list of references cited, refer to the paper published by Liu and Sherif, 2019, at Frontiers in Plant Science, https://www.frontiersin.org/articles/10.3389/fpls.2019.01408/full.
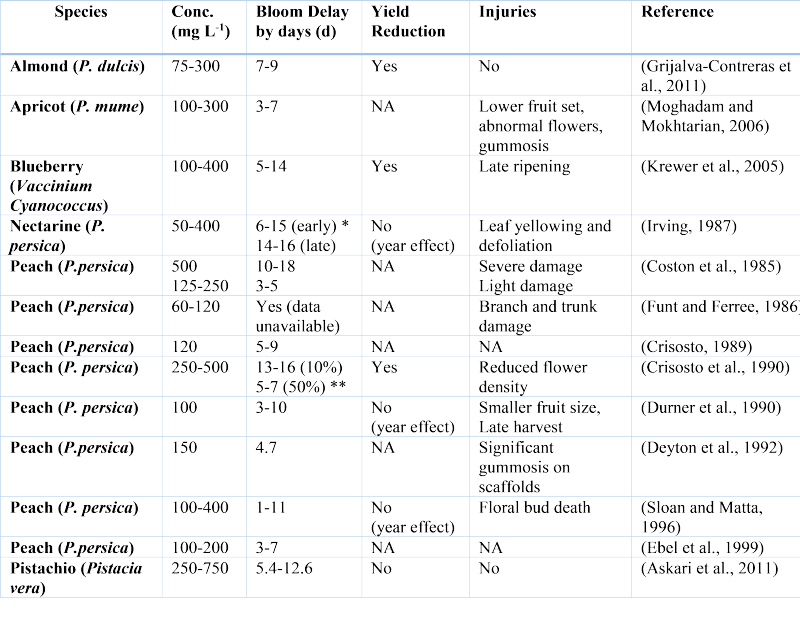
For the list of references cited, refer to the paper published by Liu and Sherif, 2019, at Frontiers in Plant Science, https://www.frontiersin.org/articles/10.3389/fpls.2019.01408/full
Figure 2: Fall applications of ethephon led to bloom delay in ‘Sun High’ peach cultivar at the AHS Jr AREC, Winchester (unpublished data). Pictures of treated and untreated trees were taken on the same day.
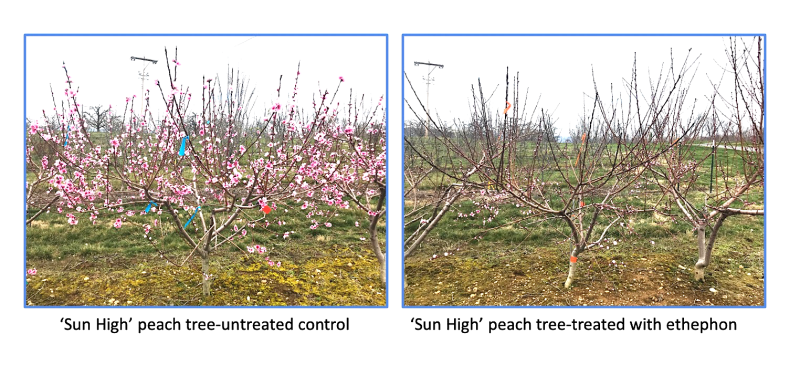